Assessment of Copper Interconnects
Enhancements in integrated circuits (IC) density and performance have fueled semiconductor industry and the resultant information revolution for over 40 years. The improvements in density (Moore’s Law) and performance has been achieved through evolutionary and revolutionary advances in the front end of the chip manufacturing line, where the circuit elements are fabricated, and the back end, where these elements are appropriately wired within the IC. Chip interconnections or interconnects, serve as global wiring, connecting circuits’ elements and distributing power.
To incorporate and accommodate the improvements such as decreased feature size, increased device speed, and more integrate designs, research in the back-end-of-the-line (BEOL) has become equally important as developing the front-end-of-line (FEOL).
Advances in interconnect technology have one important milestone in the planar multilevel metallization architecture, where planarity is achieved by the extensive use of Chemical Mechanical Polishing – CMP. Planar wiring has improved structural integrity and facilitated continuous improvements in wiring pitch, number of metal layers, and design features, such as stacked vias and local interconnections. Aluminum has been replaced with electrochemically deposited copper, and, consequently, the industry-standard subtractive metal etching process has been replaced by a damascene process: wire patterns (trenches and via holes) are first etched in an insulator, then filled with copper, and excess copper is removed by chemical mechanical planarization. In a dual damascene process both via and trench structures are filled in a single step.
Copper interconnects fabricated by a dual damascene process offer advantages of performance, cost, reliability over existing aluminum wiring process. Performance is gained because the resistivity of copper is approximately 40% lower than that of aluminum, so copper wires exhibit approximately 40% lower RC delay than aluminum wires of the same cross section. Moreover, the dual damascene approach, compared with single damascene, provides lower via resistance reducing the number of interfaces in the vias. Cost reduction comes from eliminating some process steps and simplifying other process steps in the dual-damascene process. Reliability is improved because the electrochemical deposited copper, when compared with aluminum, exhibit far less electromigration and far less stress migration.
The present work on copper interconnects resulted from an internship carried out at the Interuniversity MicroElectronics Center – IMEC -, Leuven, Belgium, a challenging international leading edge research center. The subject described in this thesis covers most of the work that I carried out from the beginning of August to the second half of November, 2005.
Four main topics were covered and they have lead to the four-chapter structure of this thesis. A brief introduction is present, too. It gives a general overview of the main process steps in the interconnect technology.
“Chapter 1: Interconnect RC delay simulations” describes an attempt to model interconnect RC delay starting from geometric considerations by comparing the simulated delay with the measured one, to identify the impact of several assumptions on the RC delay extraction accuracy. Capacitance simulations were carried out using Synopsys Raphael™.
“Chapter 2: Within-die and within-wafer interconnect R and C systematic variability” aims at understanding systematic interconnect resistance and capacitance variability both at die and wafer level. Variability at die level is dominated by layout pattern dependencies while variability at wafer level is related to process signatures.
“Chapter 3: Response Surface Modeling of interconnect capacitance” tries to correlate electrical capacitance and interconnect physical dimensions, i.e., how small variations in the interconnect cross-sectional dimensions lead to changes in the interconnect capacitance.
“Chapter 4: X-ray fluorescence assessment of interconnect thickness” aims at understanding XRF line scan measurements over patterned regions through simulations.
Two small appendices complement these four chapters.
“Appendix I: Evaluation of interconnect resistivity” describes the steps used to measure copper interconnect resistivity.
“Appendix II: XRF line-scan simulator “Deo Gratias” Matlab® code” simply contains the Matlab® code of the XRF line-scan simulator used in the study described in chapter 4.
CONSULTA INTEGRALMENTE QUESTA TESI
La consultazione è esclusivamente in formato digitale .PDF
Acquista
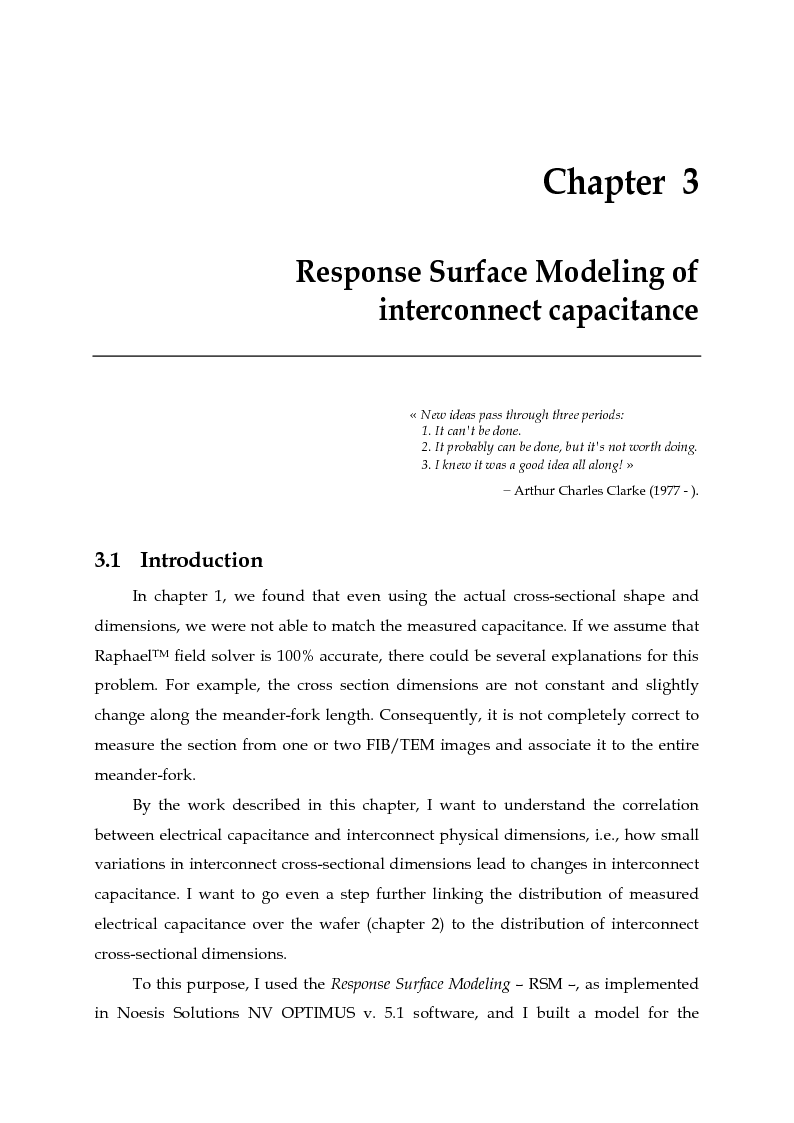
CONSULTA INTEGRALMENTE QUESTA TESI
La consultazione è esclusivamente in formato digitale .PDF
Acquista
Informazioni tesi
Autore: | Gerardo Bottiglieri |
Tipo: | Tesi di Master |
Master in | International Master in Nanotechnologies|
Anno: | 2005 |
Docente/Relatore: | Jean Paul Gueneau de Mussy |
Istituito da: | Università Ca' Foscari di Venezia e Università degli Studi di Padova |
Lingua: | Inglese |
Num. pagine: | 151 |
Forse potrebbe interessarti la tesi:
Nanotecnologia: dall'applicazione al business d'impresa
FAQ
Come consultare una tesi
Il pagamento può essere effettuato tramite carta di credito/carta prepagata, PayPal, bonifico bancario.
Confermato il pagamento si potrà consultare i file esclusivamente in formato .PDF accedendo alla propria Home Personale. Si potrà quindi procedere a salvare o stampare il file.
Maggiori informazioni
Perché consultare una tesi?
- perché affronta un singolo argomento in modo sintetico e specifico come altri testi non fanno;
- perché è un lavoro originale che si basa su una ricerca bibliografica accurata;
- perché, a differenza di altri materiali che puoi reperire online, una tesi di laurea è stata verificata da un docente universitario e dalla commissione in sede d'esame. La nostra redazione inoltre controlla prima della pubblicazione la completezza dei materiali e, dal 2009, anche l'originalità della tesi attraverso il software antiplagio Compilatio.net.
Clausole di consultazione
- L'utilizzo della consultazione integrale della tesi da parte dell'Utente che ne acquista il diritto è da considerarsi esclusivamente privato.
- Nel caso in cui l’utente che consulta la tesi volesse citarne alcune parti, dovrà inserire correttamente la fonte, come si cita un qualsiasi altro testo di riferimento bibliografico.
- L'Utente è l'unico ed esclusivo responsabile del materiale di cui acquista il diritto alla consultazione. Si impegna a non divulgare a mezzo stampa, editoria in genere, televisione, radio, Internet e/o qualsiasi altro mezzo divulgativo esistente o che venisse inventato, il contenuto della tesi che consulta o stralci della medesima. Verrà perseguito legalmente nel caso di riproduzione totale e/o parziale su qualsiasi mezzo e/o su qualsiasi supporto, nel caso di divulgazione nonché nel caso di ricavo economico derivante dallo sfruttamento del diritto acquisito.
Vuoi tradurre questa tesi?
Per raggiungerlo, è fondamentale superare la barriera rappresentata dalla lingua. Ecco perché cerchiamo persone disponibili ad effettuare la traduzione delle tesi pubblicate nel nostro sito.
Per tradurre questa tesi clicca qui »
Scopri come funziona »
DUBBI? Contattaci
Contatta la redazione a
[email protected]
Parole chiave
Tesi correlate
Non hai trovato quello che cercavi?
Abbiamo più di 45.000 Tesi di Laurea: cerca nel nostro database
Oppure consulta la sezione dedicata ad appunti universitari selezionati e pubblicati dalla nostra redazione
Ottimizza la tua ricerca:
- individua con precisione le parole chiave specifiche della tua ricerca
- elimina i termini non significativi (aggettivi, articoli, avverbi...)
- se non hai risultati amplia la ricerca con termini via via più generici (ad esempio da "anziano oncologico" a "paziente oncologico")
- utilizza la ricerca avanzata
- utilizza gli operatori booleani (and, or, "")
Idee per la tesi?
Scopri le migliori tesi scelte da noi sugli argomenti recenti
Come si scrive una tesi di laurea?
A quale cattedra chiedere la tesi? Quale sarà il docente più disponibile? Quale l'argomento più interessante per me? ...e quale quello più interessante per il mondo del lavoro?
Scarica gratuitamente la nostra guida "Come si scrive una tesi di laurea" e iscriviti alla newsletter per ricevere consigli e materiale utile.
La tesi l'ho già scritta,
ora cosa ne faccio?
La tua tesi ti ha aiutato ad ottenere quel sudato titolo di studio, ma può darti molto di più: ti differenzia dai tuoi colleghi universitari, mostra i tuoi interessi ed è un lavoro di ricerca unico, che può essere utile anche ad altri.
Il nostro consiglio è di non sprecare tutto questo lavoro:
È ora di pubblicare la tesi